INTRODUCTION
In January 2021, Alberta Energy reserved the Crown-owned pore space of the Elk Point Group in the red shaded area indicated in Figure 1B for the potential long-term storage of CO2, pre-emptively restricting all Crown dispositions of Petroleum and Natural Gas tenure to the same subsurface area indefinitely. Then, in May 2021, Alberta released Information Letter 2021-19 regarding its intention to host a competitive process for the selection of carbon sequestration hub proposals. A formal solicitation of expressions of interest for carbon sequestration hubs followed in September 2021, ahead of the request for Full Project Proposals to be issued to industry by the end of 2021. The Province’s selection of successful proposals is anticipated by the end of March 2022.
Citing economic diversification and the energy transition, the Alberta Government has separately been promoting the development of Alberta’s lithium-enriched subsurface brines and helium resources. What is not immediately obvious is that prospective carbon capture and storage (“CCS”) sites and lithium and helium resources have considerable overlap in the same geologic section of the Alberta Basin, which raises the potential for clashes between competing interests. Indeed, the risks associated with the coexistence of rights to different geologic resources in the same pore space likely provided impetus for the Province’s announcement of a new Minerals Strategy and Action Plan and introduction of Bill 82, the Mineral Resource Development Act on November 4, 2021 (“Bill 82”). Bill 82 proposes to consolidate the full lifecycle regulation of metallic and industrial mineral extraction – such as lithium extraction from brines – under the authority of the Alberta Energy Regulator, where CCS and helium are presently situated.
Both CCS and the production of critical minerals such as lithium and helium build off the technical foundations of Alberta’s oil and gas sector and have the potential to feature prominently in the Province’s energy transition. However, the tenure rights to lithium, helium, and CCS are presently administered under separate regulations, which pits the issues of competition and resource conservation against increased public interest in decarbonization and economic diversification. Bill 82 aims to address these concerns in part by coordinating tenure rights and licensed operations, the details of which will be left to regulation that has yet to be articulated. To contemplate the possible applications of this game- changing legislation, one must understand its need, born of the convergence of different resources in the same sections of the subsurface. To that end, this article reviews the geologic background of lithium, helium, and CCS resources in the Alberta Basin, and offers a perspective on their overlap in the Devonian carbonate system. Future work will examine the implications that Bill 82 could have on the coordination of tenure rights and licensed operations.
OVERLAPPING INTERESTS IN ALBERTA’S DEVONIAN CARBONATE SYSTEM
I. A Brief History of the Devonian Carbonate System
Throughout the Middle and Upper Devonian (393–359 million years ago), the Alberta Basin straddled the equator on the western edge of the North American craton and was covered by a large epicontinental sea.1 Perennially warm, tropical to subtropical climates fostered oceanic conditions favourable to the development of laterally extensive carbonate platforms and coral-sponge reef complexes (the “Devonian carbonate system”) analogous to the modern carbonate platforms and reef complexes that form the Bahamian and Turks and Caicos islands.2 Where marine shales or salts were subsequently deposited overtop of and draping Alberta’s Devonian carbonate complexes, they effectively sealed them, establishing efficient trapping mechanisms for migrating hydrocarbons that would later fill the pore space within those traps. Indeed, the first oil boom that truly kickstarted Western Canada’s oil and gas industry was triggered by the 1947 blowout of the Leduc No. 1 well, which struck oil in a reservoir along the Rimbey-Meadowbrook trend of Upper Devonian Leduc reef complexes (Fig. 1A).
Fast forward over 70 years to today: thousands of wells have been drilled into Devonian carbonate platform and reef complexes in Alberta, resulting in major discoveries of oil and gas fields throughout the Province. The public availability of data from those wells has yielded a rich subsurface dataset with which present and future generations will continue to explore for solutions to energy, natural resource, and climate issues.
Within that context, Alberta’s Devonian carbonate system has been highlighted as one of the premier targets in the world for CO2 sequestration;3 its deep aquifers are promising prospects for geothermal energy;4 its saline brines contain a host of industrial minerals – including lithium – which are of strategic importance to the energy transition;5 and many of its natural gas accumulations contain economically viable concentrations of helium gas (see Fig. 1A). Among these interests, there is strong potential for the near-term convergence of CCS, lithium, and helium proponents on the same sections of the subsurface. The balance of this section introduces the basic geologic foundation of those resources and the likelihood of their convergence within the Devonian carbonate system.
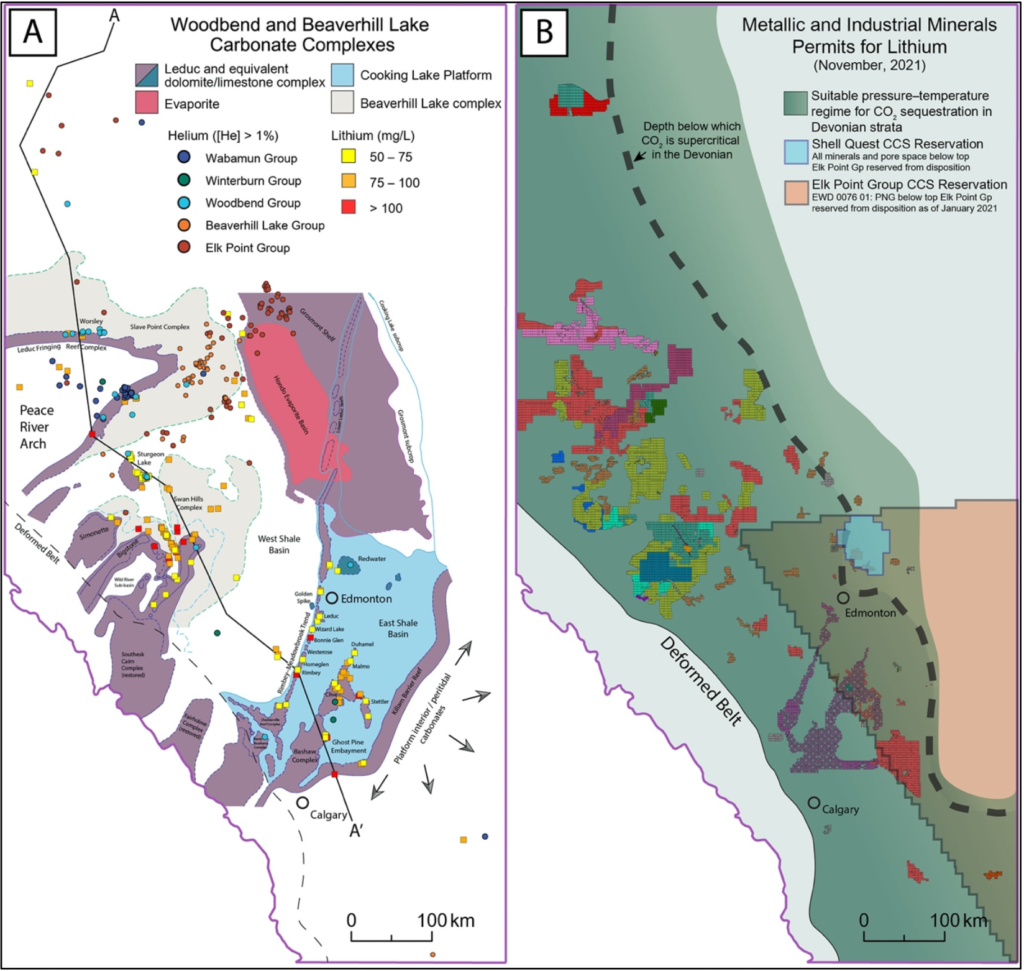
II. Alberta’s Lithium Brine Resource
Mr. Speaker, we’re also very excited about the potential for lithium here in Alberta and understand that we have some very rich lithium brines in the Devonian formations near Fox Creek, Leduc, and Swan Hills. Encouraging investment in geothermal and lithium production is important for Alberta and important to diversifying our economy, and that’s why we are taking steps to make Alberta the most competitive jurisdiction in all of North America to attract companies and innovation.6
– The Honourable Sonya Savage, Alberta Minister of Energy, June 2019.
Indeed, elevated concentrations of lithium in formation waters of the Devonian carbonate system have been known for decades and their potential economic value has been discussed for more than a quarter century.7 Lithium-enriched brines in Alberta are believed to be derived from two end-member sources. First, basinal fluids became lithium-enriched through their interaction with silicate minerals in Precambrian crystalline basement rock and the sands eroded therefrom [e.g., the Granite Wash, basal Cambrian sands, and Gilwood sandstone (Watt Mountain Formation); Fig. 2].8 The tectonic expulsion of those fluids initiated their migration through faults and permeable carrier beds into the overlying Devonian carbonate system where they enriched local formation waters with lithium.9 The Swan Hills and Slave Point reef complexes of west central Alberta and the Keg River reefs of northwest Alberta may have been particularly prone to this enrichment pathway due to their relative proximity and fault- connectedness to the crystalline basement (Fig. 2). Moreover, the migration of the same basement fluids is independently known to have caused the hydrothermal alteration (dolomitization) of several of those reef complexes, lending credence to this related model for lithium-enrichment.10
The other end-member source of lithium is the dissolution of lithium-bearing salts. During the Lower and Middle Devonian, ocean circulation in Alberta became restricted in various subbasins, enabling the repeated evapoconcentration of ion-rich seawater and the precipitation of lithium-bearing evaporite salts (e.g., the Cold Lake Salt, Black Creek Salt, Lotsberg Salt, and Prairie Evaporite; Fig. 2). It is postulated that these deeper lithium-enriched evaporite deposits were subsequently invaded by, and dissolved into, younger (Upper) Devonian seawater-derived brines, which were later pushed upward as a result of tectonism into the aquifers of the Devonian carbonate system.11

The lithium-enriched brines of the Swan Hills Formation (Beaverhill Lake Group) of west central Alberta (Fig. 1A) are believed to be derived from both end-members, while the Leduc (Woodbend Group) and Nisku (Winterburn Group) brines in southern Alberta became enriched predominantly through the dissolution of lithium-bearing salts.12 Regardless of the end-member, most, if not all of Alberta’s lithium-enriched formation brines have likely been diluted to varying degrees by meteoric water and/or other basinal fluids, such that the concentrations of lithium in those brines today are diminished relative to when they formed.13
Nonetheless, brines considered to be “enriched” in lithium (i.e., >50mg/L) are distributed throughout the Devonian carbonate system (Fig. 1A), where they co-exist with oil and gas pools and fill the pore space of the underlying saline aquifers.14 For reference, lithium recovery from brines is considered potentially economic at concentrations of 65-70 mg/L.15 The exclusive relationship between the Devonian carbonate system and Alberta’s lithium brine potential is thus highlighted by the fact that >80% of studied geochemical datapoints that can be considered lithium-enriched and >88% of those datapoints that can be considered potentially economic are from the Devonian carbonate system.16 Additionally, lithium- bearing brines in younger strata are believed to be derived from the underlying Devonian system.17 Though commercial production does not yet exist, nearly all of the known lithium-enriched brines in Alberta’s Devonian carbonate system have been staked by lithium proponents (Fig. 1B).
III. Alberta’s Helium Resource
[Establishing a royalty structure] unlocks the potential to develop helium deposits in southeastern Alberta and sets us up to take advantage of the close proximity to the United States, the world’s largest helium consumer. Economic diversification is an essential part of the Province’s recovery efforts and sets a course towards future prosperity.18
– The Honourable Sonya Savage, Alberta Minister of Energy, May 2020.
Developing untapped resources – such as helium – further diversifies our energy sector, with Alberta being well-positioned to help meet increasing global demand.19
– Jennifer Henshaw, spokesperson for Minister Savage, May 2021.
Helium: Rarer than Diamonds
The subsurface entrapment of economic quantities of helium gas depends on several of the same processes essential to a conventional hydrocarbon system: permeability pathways such as faults or carrier beds are required for migration from the source to a relatively porous and permeable reservoir, and an effective trap (reservoir closure) and seal are required for helium to accumulate. Because they share those elements, helium commonly exists as a trace component of oil and gas accumulations. The source of helium in sedimentary basins is, however, quite distinct from thermogenic cracking of organic matter or microbial sources of hydrocarbons.
Helium-4 (“4He”) is a byproduct of the radioactive decay of Uranium-238 (“238U”) and Thorium-232 (“232Th”), both of which occur naturally in certain minerals of the Earth’s crust. As these radioactive isotopes and their daughter products decay to stable Lead-206 (“206Pb”) and Lead-208, respectively, they emit alpha particles with a charge of +2. Those alpha particles pick up two electrons to become neutral 4He atoms which, unless emitted directly into the rock’s pore waters, remain within the source minerals or gradually diffuse into the surrounding pore waters. The 238U decay series yields seven atoms of 4He and 232Th produces six.20 However, the half-life of 238U (i.e., the time it takes for half of the original mass of 238U to decay into 206Pb) is approximately 4.5 billion years – roughly the age of Earth. The half- life of 232Th is approximately 14 billion years – roughly the age of the universe.
These striking numbers reflect the non-renewable nature of helium resources and the scarcity of standalone helium accumulations derived from the Earth’s crust. The likelihood of 4He accumulating in economic quantities is further dependent on there being relatively high concentrations of crustal minerals containing 238U and 232Th – such as in granite – and low porosities in the crust so that voluminous helium atoms emitted from those isotopes can concentrate in the surrounding pore waters.21 Conversely, higher porosities inhere a lower density of 238U and 232Th-bearing minerals and greater volumes of pore water to dilute the accumulation of helium.22
Where ancient, low porosity, igneous or metaigneous rocks underlie sedimentary basins, economically viable amounts of the precious gas may have had time to accumulate prior to being expelled into the overlying stratigraphy. The 1.7 to 3.27-billion-year-old western cratonic margin of the Canadian Shield underlying the Alberta Basin is one such setting.23 From there, a tectonic event(s) is generally required to heat or pressure-release the helium trapped within the mineral groundmass, and to create fractures or faults within that crystalline basement through which helium in pore waters can migrate into the overlying stratigraphy.24 Multiple compressional tectonic events likely provided such opportunities for helium release from the crystalline basement during the foreland basin stage of the Alberta Basin.
Once mobilized from the crystalline basement, helium migration through the stratigraphic column and accumulation within reservoirs is further dependent on carrier gases that enable the advection of the bulk solution from depth.25 Of particular importance is nitrogen, which is also released from crystalline basement rock through exposure to modest heat and pressure (i.e., low grade metamorphism).26 Virtually every economically viable accumulation of 4He gas in sedimentary basins is associated with nitrogen gas, highlighting its importance as an advective carrier of helium from their common source to the reservoir.27 Very high nitrogen reservoirs containing helium are known to exist in southernmost Alberta,28 where they overlie some of the oldest crust in the Province – 2.65 to 3.27 billion year-old plutonic (i.e., crystallized magmatic plume) basement.29 Such reservoirs may have retained their high nitrogen content due to a lack of interaction with migrating hydrocarbons, which in turn could be due to a lack of hydrocarbon source rock within the catchment of those reservoirs. Accumulations of nitrogen containing helium are highly sought after because, once helium is stripped from the gas, nitrogen can be safely vented to the atmosphere. A handful of dedicated helium exploration companies are poised to begin production in this region.30 Elsewhere in the Province, helium occurrences are more commonly associated with a greater proportion of methane and other short-chained hydrocarbons.
The Devonian Carbonate System: Host Reservoirs for Helium
Of the available Alberta wells with gas analyses reporting helium concentrations >0.5%, the age of the host reservoir of more than 61% of those wells is Devonian.31 Figure 1A further differentiates Devonian reservoirs hosting helium concentrations >1% between the Elk Point, Beaverhill Lake, Woodbend, Winterburn, and Wabamun Groups. The Devonian dominance is related to both the proximity of those reservoirs to the Precambrian basement source of 4He [especially the Granite Wash, Keg River Formation, Gilwood sandstone (Watt Mountain Formation), and Slave Point Formation],32 and the integrity of the marine shale and evaporite seals draping those reservoirs (e.g., Muskeg Formation, Prairie Evaporite, Woodbend/Waterways shales). The latter factor is essential due to the atomic radius of 4He, which is smaller than hydrocarbons, enabling it to leak (diffuse) through certain seals that might otherwise trap oil or gas.33
Approximately 30% of studied helium occurrences >0.5% in Alberta are from Cretaceous reservoirs in southern Alberta (south of Cold Lake, approximately). Studies of this bimodal population of helium anomalies have shown that shallow Mannville (Cretaceous) occurrences are mildly enriched with primordial Helium-3 (“3He”; which occurs naturally in the atmosphere and in the mantle), whereas deeper Devonian occurrences are virtually devoid of 3He.34 This distribution suggests that helium in Devonian and older reservoirs is almost exclusively sourced from radioactive decay of 238U and 232Th in the Precambrian crystalline basement (i.e., 4He), whereas Cretaceous reservoirs are at least partly enriched with atmospheric 3He, which was potentially introduced via meteoric groundwater during the late stages of the Laramide Orogeny.35 From an exploration standpoint, Cretaceous helium anomalies appear more discretely distributed than Devonian occurrences, possibly owing to the lower net-to-gross, fluviodeltaic to marginal marine nature of Cretaceous reservoirs in southern Alberta. As a result, stripping of helium from existing Cretaceous natural gas at a central gathering facility may be more viable than the standalone development of Cretaceous-hosted helium, whereas Devonian and older helium accumulations may offer the opportunity for standalone development.
IV. Alberta’s Carbon Sequestration Resource
The Alberta Basin is one of the premier locations globally for geologic storage of CO2 based on its relatively stable tectonic setting, abundant long-term trapping mechanisms in deep saline aquifers, and favourable hydrological flow regimes – all of which support long-term storage integrity.36 Of particular importance to the future of CCS in the Province is the Devonian carbonate system, host to several deep saline aquifers confined by thick, low permeability aquitards.37 Further, the pressure–temperature conditions in the Devonian across much of the basin are conducive to the storage of CO2 as a supercritical fluid (Fig. 1B).38 Supercritical CO2 is more dense relative to gas, thus requiring less pore volume for storage. It also inheres reduced buoyancy in saline formation waters, which makes CO2 injected as a supercritical fluid less volatile and easier to manage/monitor than in gaseous form.39
For the same reasons that Devonian carbonates have made fantastic oil and gas reservoirs, they are excellent candidates for repositories of supercritical CO2. First, the high porosity of those reservoirs that provided for elevated oil and gas saturations also enables large volumes of CO2 to be stored. Second, the relatively high permeabilities that have rendered oil and gas within those reservoirs producible also enables economically viable rates of CO2 injection. Conversely, the capacity for storage in shales or coals is presently limited by the practical considerations of storage volume and economically viable rates of injection.40 By virtue of the propensity for in-situ accumulation and growth of carbonate platform and reef complexes, the Devonian carbonate system has numerous large vertical stratigraphic features with huge CO2 storage capacity (Fig. 2). For example, the saline aquifer portion of the Leduc Redwater Reef can potentially store 1 gigaton of CO2 41 – a substantial amount compared to the 125 gigatons required to be sequestered from fossil fuel and industrial sources by 2100 under the International Energy Agency’s previously suggest pathway to avoiding global warming greater than 2oC.42
In addition to the foregoing favourable geological and geothermal characteristics, the stable hydrological flow regime of the Devonian carbonate system renders its aquifers the most attractive for CO2 injection in the Province. Devonian and older aquifers in the northwest, central, and southeast parts of the Province (the green-coloured area in Fig. 1B) are confined by regional-scale thick aquitards and aquicludes that can prevent the migration of CO2 into the overlying stratigraphy.43
While it has been noted that open saline formations bounded above by continuous sealing lithologies offer superior storage capacity to structural or stratigraphic traps with defined closures,44 the propensity for injected CO2 (and the associated pressure front) to migrate laterally in unconfined reservoirs requires that an expansive geographic area be reserved for CO2 sequestration. This is precisely the case for Shell’s Quest Project, where the Province reserved all unleased Crown mineral rights and the pore space from the Middle Devonian (top of Elk Point Group) to the basement across 40 townships exclusively for CCS.45 Quest is the only active saline aquifer storage project in Alberta and involves the injection of compressed CO2 captured from Shell’s Scotford Upgrader into a 35-46 m thick basal Cambrian sands reservoir northeast of Edmonton.46
DISCUSSION
Shell’s Quest CCS Case Study
In its 2012 approval of Shell’s Quest Project, the Energy Resources Conservation Board (now the AER) noted that, despite the pre-existence of third party petroleum and natural gas leases of lands that were subsequently reserved exclusively for CCS,47 the “[basal Cambrian sands] portion of the storage complex is devoid of hydrocarbons,” making it “extremely unlikely, given the current state of knowledge of the hydrocarbon potential, any third party would apply to drill a well into this section.”48 In other words, the regulator was comfortable reserving all rights from the top of the Elk Point Group to the basement across 40 townships for Shell’s exclusive injection of CO2 (the “Reserved Zone”) because it did not perceive any other value in the geologic resources of that area. Evidently, Shell and the regulator wanted no part in split title issues arising from the ownership of separate resources in the same pore space.
It is worth noting that, while no public water analyses with lithium concentrations are available from within the Reserved Zone, the Prairie Evaporite and Lotsberg Salt are present, and those formations are believed to be the source of lithium-enriched brines in the Leduc and Nisku formations to the south of the Reserved Zone. Assuming the Reserved Zone was devoid of any value other than for CO2 sequestration, the foregoing suggests it should nevertheless be considered an outlier in that regard.
As the oil sands and other heavy CO2-emitting sectors in Alberta reckon with the need to dramatically reduce their greenhouse gas emissions, they are turning en mass to CCS. For example, in October, 2021, Operators accounting for 90% of oilsands output announced their ambition to reduce their emissions by 21-25 megatons per decade up to 2050, largely through CCS.49 For reference, the original planned capacity of Shell’s 40-township Quest CCS project was only 27 megatons.50 Further, the demand for CCS will continue to rise dramatically as Alberta’s growing petrochemicals, fertilizer, cement, hydrogen, and other industries seek to offset their point-source emissions of CO2. Consequently, any remaining unicorn reservoirs for CO2 sequestration that have no present value for other mineral, hydrocarbon, or geothermal interests will soon be reserved for CCS, forcing the demand for additional capacity to converge on the same formations that host other economically valuable geologic resources.
Evidence that this is already happening lies in Alberta Energy’s recent reservation of the Crown-owned pore space from the top of the Elk Point Group to basement across a large swathe of southern Alberta for CCS (Fig. 1B). The corollary restriction on the disposition of Petroleum and Natural Gas tenure ostensibly precludes Alberta helium explorers from posting for lands in the reserved area, notwithstanding the fact that Saskatchewan helium explorers have been making discoveries and producing helium from the equivalent zone directly across the border.
In response to questions about how it would accommodate the application of any existing rights holder to drill within the Reserved Zone at Quest, Shell submitted that “the existing process and protocols under Directive 056 should be sufficient to ensure communication between parties.”51 Directive 056 sets out the license application requirements for energy development projects, including the drilling and operation of CO2 injection wells and, if Bill 82 is enacted, it would also cover metallic and industrial mineral extraction operations. With reference to the above Shell comment, Directive 056 also lays out a “Participant Involvement” protocol for the engagement of all other mineral and leasehold interest holders, including a framework for addressing concerns/objections and alternative dispute resolution for the coordination of licensed operations in the same area of the subsurface. However, the disposition of tenure rights to the geologic resources of a specific pore space necessarily precedes the regulatory approval of licensed operations therein and the application of Directive 056, which raises the question of whether this regulatory instrument is adequately suited to preserving the interests of multiple stakeholders. More to the point, Directive 056 can’t apply to prospective future licensed activities in areas where the Province has pre-emptively restricted the disposition of tenure rights related to such activities.
As two or more interests in CCS, lithium, helium, geothermal, or oil & gas converge on the same pore space – such as in the Devonian carbonate system – the regulator will be put in the uncomfortable position of picking and choosing which projects are in the public interest. Weighing the need for resource and energy sector diversification, the economic value of competing resource development, job creation, conservation of resource requirements, public demand for better environmental stewardship, and taxpayer accountability will not be an easy undertaking. And, if valuable pore space such as in the Devonian carbonate system is to be monopolized for one purpose, as in the Shell Quest CCS scenario, interested stakeholders need to pre-emptively provide the regulator with an informed, fulsome picture of the opportunity cost of doing so (including the value of alternative uses), so that the regulator can equitably coordinate tenure rights and/or licensed operations for the conservational use of the subsurface by multiple industries. Our ongoing work will examine how such coordination may be shaped by Bill 82 and the existing regulatory regime.
Nick Ettinger, BSc (Queen’s University), MSc (University of Texas at Austin), JD (2022, University of Calgary). Nick is a geologist and future lawyer who aims to apply his background in earth sciences to the intersection of natural resources, energy, and environmental law and policy.
1 Paul Copper & Christopher R Scotese, “Megareefs in Middle Devonian supergreenhouse climates” in Extreme Depositional Environments: Mega End Members in Geologic Time Special Paper 370 (Geological Society of America, 2003) 209 at 212.
2 See ibid at 212–214.
3 Suitability of the Alberta Subsurface for Carbon-Dioxide Sequestration in Geological Media, by Stefan Bachu et al, Earth Sciences Report 2000-11 (Alberta Energy and Utilities Board, 2000) at 77–79; WD Gunter et al, “Heartland Area Redwater reef saline aquifer CO2 storage project” (2009) 1 Energy Procedia 3943–3950; Karsten Michael et al, “Comprehensive Characterization of a Potential Site for CO2 Geological Storage in Central Alberta, Canada” in Carbon dioxide sequestration in geological media — State of the science AAPG Studies in Geology 59 (AAPG Special Volumes, 2009) 227.
4 See generally, Leandra M Weydt et al, Geothermal Assessment of Two Regionally Extensive Upper Devonian Carbonate Aquifers in Alberta, Canada (Reykjavik, Iceland, 2021).
5 See Industrial Mineral Potential of Alberta Formation Waters, by B Hitchon et al, Canada Alberta Partnership on Minerals Bulletin No. 62 (Alberta Research Council, 1995); R Tscherning & B Chapman, “Navigating the emerging lithium rush: lithium extraction from brines for clean-tech battery storage technologies” (2021) 39 Journal of Energy and Natural Resources Law 13–42.
6 Alberta Legislative Assembly, Hansard, 30th Leg, 1st Sess No 14, (17 June 2019) at 821.
7 See B Hitchon et al, supra note 5 at 58.
8 Geological Introduction to Lithium-Rich Formation Water with Emphasis on the Fox Creek Area of West-Central Alberta (NTS 83F and 83K), by DR Eccles & H Berhane, ERCB/AGS Open File Report 2011-10 (Energy Resources Conservation Board, 2011) at 14; GF Huff, “Evolution of Li-enriched oilfield brines in Devonian carbonates of the south-central Alberta Basin, Canada” (2016) 64 Bulletin of Canadian Petroleum Geology 438–448 at 441.
9 DR Eccles & H Berhane, supra note 8 at 14.
10 See generally, JP Duggan, EW Mountjoy, & LD Stasiuk, “Fault-controlled dolomitization at Swan Hills Simonette oil field (Devonian), deep basin west-central Alberta, Canada” 48 Sedimentology 301–323.
11 GF Huff, supra note 8 at 446.
12 Origin and Li-enrichment of selected oilfield brines in the Alberta Basin, Canada, by GF Huff, Open File Report 2019- 01 (Alberta Geological Survey / Alberta Energy Regulator, 2019) at 13.
13 GF Huff, supra note 8 at 446.
14 Geological Introduction to E3 Metals Corp. Clearwater and Exshaw Lithium-Brine Properties in South-Central Alberta, National Instrument 43-101 Technical Report, by R Eccles, National Instrument 43-101 Technical Report (Apex Geoscience Ltd., 2017) at 2.
15 Pamela Daitch, Lithium extraction from oilfield brine (Combined MSc/MBA Thesis, University of Texas at Austin, 2018) [unpublished] at 38.
16 Lithium Groundwater and Formation-Water Geochemical Data, by DR Eccles & GM Jean, DIG 2010-0001 (Energy Resources Conservation Board / Alberta Geological Survey, 2010).
17 GF Huff, supra note 12 at 10.
18 Government of Alberta, “Press Release: New royalty rate responds to soaring helium interest”, online: <https://www.alberta.ca/news.aspx>.
19 Amanda Stephenson, “‘Land rush’ on in Alberta as demand for lighter-than-air helium takes flight”, Calgary Herald (2021), online: <https://calgaryherald.com/business/local-business/land-rush-on-in-alberta-as-demand-for-lighter-than-air- helium-takes-flight>.
20 E Grynia & PJ Griffin, “Helium in Natural Gas – Occurrence and Production” (2016) 1 Journal of Natural Gas Engineering 163–215 at 164.
21 Ibid.
22 Ibid.
23 GM Ross et al, “Geophysics and geochronology of the crystalline basement of the Alberta Basin, western Canada” (1991) 28 Canadian Journal of Earth Sciences 512–522 at 513.
24 I Hutcheon, “Controls on the distribution of non-hydrocarbon gases in the Alberta Basin” (1999) 47 Bulletin of Canadian Petroleum Geology 573–593 at 589; E Grynia & PJ Griffin, supra note 20 at 164.
25 D Danabalan, Helium: Exploration Methodology for a Strategic Resource Durham University, 2017) [unpublished] at 80.
26 CJ Ballentine & BS Lollar, “Regional groundwater focusing of nitrogen and noble gases into the Hugoton-Panhandle giant gas field, USA” (2002) 66 Geochemica et Cosmochimica Acta 2483–2497 at 2492
27 E Grynia & PJ Griffin, supra note 20 at 164.
28 The Steveville Structure and the Knappen Dome and related structures.
29 GM Ross et al, supra note 23 at 513.
30 “Knappen Asset”, online: Thor Resources <https://www.thorres.com/knappen>; “Steveville Asset”, online: Imperial Helium <https://imperialhelium.ca/about/>.
31 n = 1395; 29.8% Cretaceous, <1% Jurassic, 0.78% Triassic, 6.3% Permian-Carboniferous, 61.2% Devonian, 1% Precambrian.
32 See I Hutcheon, supra note 24 at 588: “[T]he amount of 4He increases exponentially with proximity to the Precambrian basement.”
33 D Danabalan, supra note 25 at 37.
34 See generally, H Hiyagon & BM Kennedy, “Noble gases in CH4-rich gas fields, Alberta, Canada” (1992) 56 Geochimica et Cosmochimica Acta 1569–1589; I Hutcheon, supra note 24 at 588.
35 I Hutcheon, supra note 24 at 589.
36 See generally, Stefan Bachu et al, supra note 3.
37 Ibid at 13.
38 Ibid at 21.
39 Ibid at 10–11; National Academies of Sciences, Engineering, and Medicine, “Sequestration of Supercritical CO2 in Deep Sedimentary Geological Formations” in Negative Emissions Technologies and Reliable Sequestration: A Research Agenda (The National Academies Press, 2019) 319 at 319.
40 National Academies of Sciences, Engineering, and Medicine, supra note 39 at 320.
41 WD Gunter et al, supra note 3 at 3948.
42 See National Academies of Sciences, Engineering, and Medicine, supra note 39 at 321.
43 Stefan Bachu et al, supra note 3 at 77–79.
44 ML Couëslan, Wade Zaluski, & Brody Loster, “Risk-Based Design of Site Characterization and Measurement, Monitoring, and Verification Plans for Carbon Capture, Utilization, and Storage Projects” (2021) 46 CSEG Recorder, online: <https://www.csegrecorder.com/articles/view/risk-based-design-of-site-characterization-and-measurement- monitoring> at 2.
45 Shell Canada Limited Application for the Quest Carbon Capture and Storage Project, Radway Field, Decision 2012 ABERCB 008 at para 180.
46 Ibid at para 115.
47 Ibid at para 179.
48 Ibid at para 180.
49 “Press Release: Oil Sands Pathways alliance outlines three-phase plan to achieve goal of net zero emissions”, (2021), online: Oil Sands Pathways to Net Zero <https://www.oilsandspathways.ca/pressrelease_oct21/>.
50 Shell Canada Limited Application for the Quest Carbon Capture and Storage Project, Radway Field, supra note 45 at para 167.
51 Ibid at para 124.